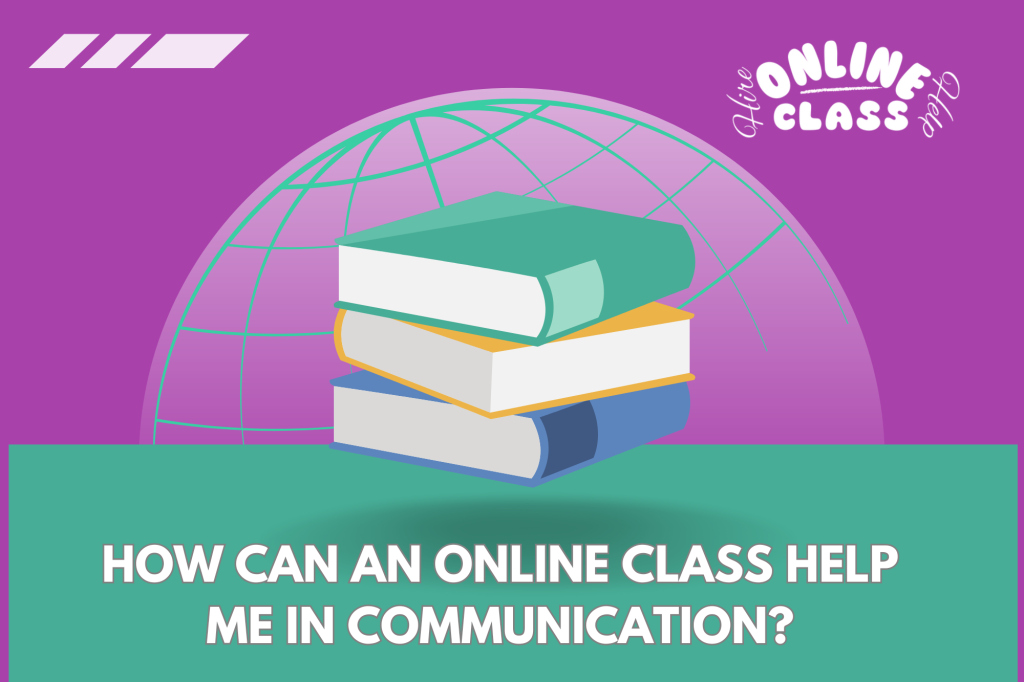
Name
Chamberlain University
BIOS-256 Anatomy & Physiology IV with Lab
Prof. Name
Date
In the previous section, we explored the DNA within gametes, which are organized into 23 haploid chromosomes, and examined how they contribute to the genetic diversity of offspring. This section seeks to answer several questions, such as why children resemble their parents and how they inherit familial diseases. A gene is defined as a short segment of DNA located on a chromosome, with many genes present along its length. Each chromosome is a long strand of DNA that can contain hundreds to thousands of genes. Genes provide instructions for the cell to synthesize proteins based on the specific sequences in DNA. Chromatin encompasses all the DNA in the nucleus that is not coiled into chromosomes.
Gametes, unlike normal body cells, contain only 23 single chromosomes without homologous pairs. Normal body cells have 23 homologous pairs of chromosomes, with one pair being sex chromosomes and the remaining 22 being autosomes. The genetic vocabulary presented through karyotypes illustrates that chromosomes vary in size and may consist of a few hundred to several thousand genes. Homologous chromosomes possess the same genes at identical positions; however, the gene on the maternally derived chromosome may differ in form from the gene on the paternal chromosome. These variations of the same gene are referred to as alleles. Typically, one allele is dominant (indicated by a capital letter), while the other is recessive (indicated by a lowercase letter). Since individuals have two chromosomes in each homologous pair, they possess two alleles for each gene. If both alleles are dominant (AA), the individual is termed homozygous dominant; if both are recessive (aa), the individual is homozygous recessive; and if the alleles are one of each (Aa), the individual is heterozygous. The collection of alleles that an individual carries for a specific gene is known as the genotype, whereas the observable physical trait is referred to as the phenotype.
A dominant allele will manifest in the phenotype if at least one copy is present, effectively masking the presence of a recessive allele, which requires two copies to be expressed. For example, the cleft chin gene exhibits a dominant-recessive inheritance pattern where the cleft chin trait (A) dominates over the uncleft chin trait (a). Consequently, individuals with a genotype of Aa or AA express the cleft chin phenotype, while those with the homozygous recessive genotype (aa) do not.
In some instances, there exist more than two allele options for a single gene. The human blood typing system is a notable example, where three alleles—IA, IB, and i—determine blood type. In this system, IA and IB are both dominant and co-expressed, while i is recessive. In cases of incomplete dominance, the phenotype is an intermediate of the two alleles, such as with sickle cell anemia. Individuals who are homozygous for the sickle cell allele experience the full condition, while heterozygotes exhibit a milder form.
Traits such as cleft chin, widow’s peak, and Huntington’s disease are examples of traits governed by a single gene, termed monogenic inheritance. In contrast, most human traits result from the interaction of multiple genes across various chromosomes, known as polygenic inheritance. Environmental factors also play a role in how these traits are expressed. Examples of polygenic traits include eye color, height, skin color, and susceptibility to diseases like diabetes and cancer.
Examples of Monogenic Traits | Examples of Polygenic Traits |
---|---|
Albinism (lack of skin pigment) | Metabolism |
Polydactyly (extra digits) | Diabetes mellitus |
Huntington disease | Body shape |
Cystic Fibrosis | Height |
Diabetes insipidus | Skin color |
Syndactylism (webbed digits) | Eye color |
Widow’s peak (hairline) | Mental illness |
Height exemplifies a polygenic trait controlled by multiple genes, with environmental influences, particularly nutrition, also playing a significant role in its expression.
While the inheritance of genetic factors from parents to offspring has been understood for over a century, the study of nongenetic factors that influence inheritance, termed epigenetics, is relatively new. Epigenetics investigates the mechanisms governing gene expression—how genes are activated or silenced in offspring. Although the human genome contains approximately 30,000 genes, only a few hundred are active at any given time, with the rest being turned off through processes such as DNA methylation, which involves adding methyl groups (CH3) to the DNA. Lifestyle choices and environmental conditions can lead to incorrect methylation in gametes, affecting gene expression that may be passed to offspring.
Epigenetics represents a significant field of study that explores how genes are expressed without altering the DNA sequence directly. It involves various mechanisms that regulate gene expression, ultimately affecting the proteins produced by cells.
The X and Y sex chromosomes not only determine an individual’s sex but also carry essential genes beyond reproduction. The X chromosome contains hundreds of non-reproductive genes necessary for survival, while the Y chromosome has fewer genes primarily associated with male reproduction. Females possess two X chromosomes, providing two alleles for each X-linked gene, whereas males have only one X and therefore one allele, rendering them hemizygous for these genes. Mutations in X-linked genes can lead to various recessive disorders, such as color blindness, hemophilia, and muscular dystrophy. Females require two recessive alleles to express these conditions, while males only need one, making them disproportionately affected by sex-linked recessive disorders.
Fathers pass their X chromosome to daughters and Y chromosome to sons, while mothers provide an X chromosome to each child. If a mother is a carrier for color blindness (genotype XC Xc), she does not exhibit the condition due to the presence of a normal dominant allele but can pass the recessive allele (Xc) to her sons, leading to a 50% chance of color blindness. Sons receive a Y chromosome from their father, which does not carry the color blindness allele, while daughters inherit an X chromosome from both parents.
Postnatal Genetic Testing: Postnatal genetic testing may be performed if a child fails to meet standard developmental milestones. Various genetic tests are available to check for missing or duplicated chromosome segments, translocations, or specific gene mutations. Multiple tests may be necessary to reach a diagnosis, and genetic counselors can assist in selecting appropriate tests and interpreting results.
Karyotype: A karyotype analysis, which can be conducted pre- or postnatally, identifies chromosomal abnormalities, although more sensitive molecular genetic testing methods are now available.
Safest Tests for the Fetus: The safest testing options include:
CVS and amniocentesis carry a small risk of miscarriage (<2%) due to their invasive nature.
In summary, the genotype comprises two of the three possible alleles, with IA and IB expressed equally in the phenotype, classified as codominant. The recessive allele, i, leads to a Type O phenotype when two copies are inherited. X-linked disorders predominantly involve genes located on the X chromosome, as the Y chromosome carries few essential genes.
If diabetes mellitus is prevalent in a family, genetic testing may reveal multiple genes that contribute to the condition, indicating a polygenic inheritance pattern influenced by environmental factors. A carrier genotype example is XHXh, which denotes a female carrier (heterozygous), who does not express the recessive condition due to the presence of a dominant allele. Males (XY) cannot be carriers for X-linked conditions, as they only possess one allele.
In the case of a colorblind man, he cannot blame his father for the defective gene since he inherited his Y chromosome from him, which does not carry the gene for color blindness. His mother, being a carrier, contributed the affected X chromosome. Heterozygous individuals for the sickle cell trait exhibit an incomplete dominance inheritance pattern, where both alleles are expressed, resulting in a phenotype that lies between normal red blood cells and those affected by sickle cell anemia. The pair of homologous chromosomes displayed shows that they share the same genes at identical locations, confirming their homologous nature.
Get In touch
Let's Connect: We're Here to Help You Succeed!
Have a question or need support? Connect with our team today. We’re ready to assist you with personalized guidance to help you achieve your academic goals. Reach out via email, phone, or our easy-to-use contact form.
For urgent help
+1 (571) 899-4759
Mail us 24/7
info@hireonlineclasshelp.com
Get expert assistance to excel in your courses with personalized support. Our creative approach ensures your academic success every step of the way.
Our Services
Copyright © 2024 hireclassonlinehelp.com All Rights Reserved.